Audiology & Neuroscience | July 2018 Hearing Review
Observing changes in brain processing–and adjusting our intervention strategies accordingly
With a better understanding of cortical brain changes associated with hearing loss, the potential to develop objective brain-based tools (ie, biomarkers) increases. These tools may help clinicians determine when a patient should receive intervention, what kind of intervention or rehabilitation would be ideal, and may offer the ability to monitor how well a chosen intervention or rehabilitation method is working.
How does the brain compensate for hearing loss?
One of the most interesting aspects of the brain is its ability to adapt and to change. The term neuroplasticity refers to changes in neural connections, pathways, and networks as a result of maturation and development, sensory deprivation, injury, disease, dysfunction, and learning. Neuroplasticity is a process which occurs at all levels of the neural pathways and throughout the entire lifespan. As hearing care professionals—whether our jobs involve activating a cochlear implant, fitting a hearing aid, or providing rehabilitation to adults or children to help them reach their optimum performance after intervention—neuroplasticity is at the heart of what we do.
Electroencephalography (EEG) provides an inexpensive and non-invasive way to assess neuroplasticity in patients with hearing loss, and the auditory brainstem response (ABR) offers insight into neuroplasticity, too. That is, we can examine the function of higher auditory centers of the brain (eg, auditory cortex) using cortical auditory evoked potentials (CAEPs).
In this review, we will discuss how CAEPs can be used to assess development of the auditory cortex and monitor the maturation of the auditory cortex and central auditory pathways before and after intervention with hearing aids and cochlear implants. In addition, we will describe how the other senses compensate for hearing loss via a process known as cross-modal reorganization, and we’ll address how these brain changes are linked to real-world clinical outcomes, such as speech perception.
As a profession, we’re just beginning to unearth the widespread central effects of hearing loss, including its effect on cognitive function, listening effort, and social-emotional changes. With a better understanding of cortical brain changes associated with hearing loss, the potential to develop objective brain-based tools (ie, biomarkers) increases. These tools may help clinicians determine when a patient should receive intervention, what kind of intervention or rehabilitation would be ideal, and may offer the ability to monitor how well a chosen intervention or rehabilitation method is working.
The P1 Cortical Auditory Evoked Potential As A Biomarker
The cortical auditory evoked potential (CAEP) response is comprised of three parts: the P1, N1, and P2. The P1 response is the first positive peak in the response. The P1 response is present at birth, occurring around 300 milliseconds post-stimulation. As a child grows and their auditory system becomes more efficient, the P1 response decreases systematically in latency until it reaches 50-70 milliseconds in adulthood. Because the P1 response changes as a function of age, it can be used as an objective biomarker of auditory cortex maturation. The later CAEP components, the N1 and P2, emerge in later childhood and adolescence, and reflect higher levels of auditory processing.1-6
We have collected P1 responses in hundreds of normal-hearing children, allowing us to establish norms or “confidence intervals” for normal P1 latency. This allows us to compare responses from individual infants and children with hearing loss to typically developing children of the same age in order to determine whether auditory cortical development is normal, delayed, or abnormal (absent).7
Our research and research by others led to the discovery of a 3.5-year central auditory sensitive period, or time period during which the brain is maximally “plastic.”4,7-10 Based on this research, we know that early cochlear implantation within this sensitive period (best by age 1) leads to more normal brain development and yields more optimal listening and spoken language outcomes compared to late cochlear implantation.7,11-15 For example, most children who receive an implant within this 3.5-year time window develop normal P1 responses, whereas late-implanted children continue to show delayed P1 responses.7
Clinical Power of the P1 Biomarker in Complex Pediatric Cases
Unfortunately, not all clinical cases are clear-cut. In pediatrics, an estimated 30-40% of children with hearing loss have additional physical, developmental, or cognitive disabilities.16-19 For example, auditory neuropathy spectrum disorder (ANSD) is a special population in which the P1 biomarker may be useful. ANSD is estimated to account for 5-15% of children with sensorineural hearing loss.20-23 ANSD refers to an irregular or “dyssynchronous” firing of auditory neurons at the level of the inner hair cells and auditory nerve.
Clinically, ANSD is diagnosed based on several characteristics: Present outer hair cell function (present otoacoustic emissions and cochlear microphonic), abnormal auditory pathway function (absent or elevated acoustic reflex thresholds (ART), and an abnormal ABR response).24 One of the most challenging characteristics of ANSD is its variable clinical presentation. Children with ANSD may present with normal, temporary, fluctuating, or mild to profound hearing loss on conventional audiometric tests. Often, the audiogram doesn’t reflect the degree of impairment a child with ANSD has, with regard to understanding complex signals such as speech.20,25-26 Therefore, it can be difficult for an audiologist to obtain complete, reliable behavioral information when a child presents with ANSD.
Intervention with hearing aids and/or cochlear implants often occurs later in children with ANSD than for children with hearing loss and no other associated disabilities.27,28 Even after intervention with hearing aids or cochlear implants, children with multiple disabilities may progress at slower rates, and their skills may progress on widely varying trajectories.16-19 Further, with regard to “sedated ABRs” obtained under general anesthesia, these procedures may pose a serious risk in children with severe health conditions, rendering sedated ABR impractical in some situations.29,30 For these reasons, the P1 biomarker may serve as a valuable clinical tool to evaluate whether a child is receiving sufficient auditory input and to monitor a child’s progress after intervention.7-10,31-36 Because the P1 response comes from the auditory cortex, we can get a more holistic view about how auditory information is being processed throughout their auditory system.
P1 responses can be easily recorded on most clinical EEG systems with just a few additional electrodes.32 (However, additional methods are needed for children with cochlear implants to minimize the scalp-recorded cochlear implant artifact37). Unlike ABR, the child remains awake during P1 testing. However, the child’s attention is distracted by watching a video without sound, thereby avoiding the need for sleep or sedation.
The following cases illuminate how the P1 biomarker can be used in complex situations to determine when intervention is necessary, what kind of intervention is appropriate, and how effective a chosen intervention is working.
Case 1: A child born full term. Use of supplemental oxygen was required during this child’s birth. The child failed his newborn hearing screening bilaterally and was diagnosed with ANSD before age 3 months.
Case 1 was referred to our laboratory for P1 testing before and after intervention with bilateral hearing aids. Figure 1a depicts P1 CAEP waveforms prior to hearing-aid fitting. A robust and replicable P1 response was present; however, when plotted against 95% confidence intervals for normal P1 latency development, the child’s response was delayed for his age, indicating delayed cortical auditory maturation (Figure 1c).
Figures 1a-c. P1 CAEP waveforms (with replications) for an ANSD child in prior to hearing aid use (Panel A) and several months after bilateral hearing aid use (Panel B). Averaged P1 CAEP latencies in the unaided and aided conditions are plotted against 95% confidence intervals for normal P1 latency9 (Panel C). Results indicate delayed P1 latency prior to hearing aid use. Within several months of hearing aid use, P1 latency was age-appropriate. Adapted from Cardon et al (2012).36
At age 0.68 years, the child received bilateral hearing aids. Figure 1b depicts the child’s P1 CAEP waveforms after several months of hearing aid use. After intervention, a robust and replicable P1 response could be observed, and the latency of this response fell within normal limits for the child’s age (Figure 1c), consistent with the child’s progress in listening and spoken language after hearing aid fitting.
These results demonstrate how the P1 biomarker can be used as an objective tool to determine when audiological intervention is appropriate, and to monitor how well a chosen intervention method is working.36
Case 2: A child born premature. The child, born premature, presented with several high-risk factors for hearing loss, including a stay in the neonatal intensive care unit, hyperbilirubinemia requiring blood transfusion, and prolonged mechanical ventilation. The child failed his newborn hearing screening and was diagnosed with ANSD before age 3 months.
The child was fit with bilateral hearing aids at age 0.36 years. Case 2 was referred for P1 testing in our laboratory due to concerns about delays in speech and language development. We assessed the child’s P1 response at two different test intervals (ages 0.67 years and 1.36 years) during hearing aid use. Figure 2a depicts P1 CAEP waveforms at one of these aided test sessions. As can be seen, no replicable P1 response could be observed, indicating that the hearing aids were providing inadequate auditory input to allow for normal auditory cortical maturation.
Figures 2a-c. P1 CAEP waveforms (with replications) for an ANSD child during bilateral hearing aid use (Panel A) and several months after cochlear implantation of the left ear (Panel B). Averaged P1 CAEP latencies hearing aid and cochlear implant conditions are plotted against 95% confidence intervals for normal P1 latency9 (Panel C). Results indicate absent P1 responses during hearing aid use. Within several months after left-ear cochlear implantation, P1 latency was age-appropriate. Adapted from Cardon at al (2012).36
At age 1.76, the child received a cochlear implant in the left ear. Figure 2b shows the child’s P1 CAEP waveforms after several months of implant use. Cochlear implant results showed P1 latencies that fell within the 95% confidence intervals for the child’s age, indicating age-appropriate development of the central auditory pathways. In this case, electrical stimulation from the cochlear implant appeared to provide rapid improvement, leading to age-appropriate auditory cortical development.36
Case 2 highlights how the P1 biomarker may be used to determine what kind of intervention method may be ideal in complex pediatric cases.
Beyond the Auditory System: How Other Senses Compensate in the Presence of Hearing Loss
Given substantial hearing loss, when auditory cortical areas of the brain do not receive normal input (eg, the input is either decreased and/or degraded), areas of the brain which normally process sound may be “repurposed” by other intact modalities. Indeed, visual and vibrotactile modalities sometimes facilitate a re-assignment of “unused auditory anatomy” for their own purposes. This process is referred to as cross-modal neuroplasticity.38-50
Animal studies suggest that as a result of cross-modal neuroplasticity in hearing loss, certain kinds of perception in the recruiting modality (eg, vision) are enhanced.38 In our laboratory, we employ EEG to record cortical activity in response to auditory, visual, and vibrotactile stimuli to examine cortical brain changes across different sensory modalities to determine the extent of cross-modal recruitment in hearing loss. We use this information to assist in clinical decision making as highlighted in the case examples below.
How Does the Brain Change in Single-Sided Deafness?
While it was previously thought that cross-modal reorganization was limited to adults and children with complete deafness, cross-modal reorganization can occur in lesser degrees of hearing loss, and in adults and children with unilateral hearing loss.43,51 Despite having one good hearing ear, patients with single-sided deafness (SSD) experience many functional problems, such as difficulty understanding speech in background noise and difficulty localizing sounds.52 Research indicates children with SSD are at increased risk for speech and language delays, as well as educational delays and behavior problems.53 Cochlear implants are emerging as a new treatment option for SSD.54
Figure 3 shows brain activation patterns in response to visual, auditory, and somatosensory stimuli from a child with single-sided deafness, before and after receiving a cochlear implant.43 The child was late identified with normal hearing in the left ear and a moderate sensorineural hearing loss in the right ear at age 5. By age 9, her hearing loss in the right ear progressed to severe-profound. At approximately age 10, she received a cochlear implant (CI) in her right ear. Figure 3a (left panel), shows that, prior to receiving the CI, visual stimulation resulted in activation of the visual cortex andactivation of the auditory cortex. This unusual activity (auditory cortex activation from visual stimuli) suggests cross-modal reorganization by vision.
Figures 3a-c. Cortical visual, somatosensory, and auditory neuroplasticity in a pediatric case of single-sided deafness before and after cochlear implantation. Before cochlear implantation, the auditory cortex is recruited for visual processing (Panel A) and somatosensory processing (Panel B), suggestive of cross-modal reorganization. Post-implantation, complete or partial reduction in auditory cortex recruitment for visual and somatosensory processing is observed. Before cochlear implantation, auditory stimulation results in abnormal (eg, ipsilateral) activation of the auditory cortex (Panel C), as well as the additional recruitment of frontal and prefrontal cortex—indicative of effortful listening. Post-implantation, a restoration of more typical (eg, contralateral) auditory cortical activation and reduction in frontal activation is observed. Adapted from Sharma et al (2016).43
Similarly, somatosensory stimulation resulted in not just somatosensory regions of the brain, but also auditory cortical areas, suggestive of cross-modal reorganization by somatosensation (Figure 3b, left panel).
We observed other forms of abnormal compensation in this child. For example, as shown in Figure 3c (left panel), when we presented auditory stimuli, only the ipsilateral auditory cortex was activated, and surprisingly, frontal and prefrontal areas of the brain were activated. This frontal cortex activation may indicate “effortful” listening, where the brain has to recruit additional resources to process auditory information in the presence of hearing loss. This frontal cortex activation has been observed in other clinical populations with hearing loss and in normal-hearing adults under difficult listening conditions, such as in background noise.55-57
Interestingly, within 27 months of cochlear implant use, we observed partial reversal of cross-modal recruitment of the auditory cortex by vision (Figure 3a, right panel), complete reversal of cross-modal recruitment of auditory cortex by somatosensory modality (Figure 3b, right panel), and more typical auditory (eg, contralateral) patterns of activation (Figure 3c, right panel). Of note, there was no longer any frontal cortex recruitment for auditory processing, suggestive of less effortful listening.
The restoration of more typical sensory processing patterns after cochlear implantation was consistent with remarkable improvements in the child’s speech perception performance after receiving her CI. Within several years after cochlear implantation, this child showed localization abilities comparable to normal-hearing adults, and speech perception in noise performance comparable with other adult cochlear implant users.43 It is likely that the small amount of auditory cortex activation we see for visual processing reflects the child’s continued dependence on visual cues for some speech perception, which is common in children with CIs.58
Does Mild-Moderate Hearing Loss Result in Brain Changes? Is Treatment with Hearing Aids Beneficial?
We have observed changes in cortical neuroplasticity in adults with early-stage, mild-moderate hearing loss.42-55 That is, even in the early stages of hearing loss, the brain appears to adapt and reorganize. In mild-to-moderate hearing loss, the auditory cortex shows decreased stimulation by sound, resulting in effortful listening, as shown by frontal cortex activation. These listeners compensate by relying more on visual cues, which likely helps promote the cross-modal recruitment by vision that we observe.42,46-49,55 Interestingly, these changes may occur as soon as 3 monthsafter the onset of hearing loss and may be reversible by well-fitted hearing aids.39
We are in the early stages of our studies on whether and how brain changes might be reversed by hearing-aid use. Preliminary results are promising. Figure 4 depicts cortical brain patterns in a single case study of an adult male with mild-to-moderate hearing loss. We examined his cortical activation patterns in response to visual stimulation before and after intervention with bilateral hearing aids. As seen in Figure 4a, prior to hearing aid fitting, visual stimulation elicited activation of both visual cortical regions and auditory cortical regions, suggesting cross-modal reorganization by vision.
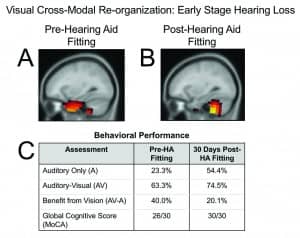
Figures 4a-c. Cross-modal recruitment by vision in mild-moderate bilateral sensorineural hearing loss before and several weeks after hearing-aid fitting. Panel A: Pre-hearing aid fitting, the adult shows recruitment of temporal (auditory) cortex for visual processing in addition to occipital (visual) cortical areas, suggestive of cross-modal reorganization by vision. Panel B: Within several weeks of hearing aid use, we see a reversal of cross-modal recruitment and expected activation of cerebellar/occipital areas to a visual motion stimulus. Panel C: Behavioral performance on an auditory/visual speech perception in noise test (AzAV) delivered as auditory-only and auditory-visual conditions, and a global cognitive function assessment (MoCA) administered pre-hearing aid fitting and post-hearing aid fitting. Within several weeks of hearing-aid fitting, this adult showed increased auditory-only performance in background noise, decreased functional reliance on visual cues for speech perception, and increased global cognitive function.
However, within several weeks of hearing-aid fitting, the cross-modal recruitment of auditory cortex by vision reversed (Figure 4b). Correspondingly, this adult showed increased auditory performance in background noise, decreased dependence on visual cues for speech perception, and increased global cognitive function after hearing aid use (Figure 4c). While this is data from a single case study and should be interpreted with caution, our laboratory is continuing to conduct larger group studies in adults with age-related hearing loss before and after the use of well-fitted amplification.
Brain reorganization may also provide an explanation for the differences in outcomes that we see in long-term hearing aid users. Figure 5 highlights differences in cross-modal reorganization between two adult hearing aid users with similar hearing loss, but different clinical outcomes.39 Both adults had a mild-to-moderate hearing loss and had worn bilateral hearing aids for at least 18 months. Figure 5a shows expected activation to visual stimuli (and no evidence of cross-modal recruitment) in the adult who demonstrated excellent speech perception in noise. In contrast, Figure 5b demonstrates cross-modal recruitment of auditory cortical areas by vision in an adult patient who demonstrated poor speech perception in noise.
Figures 5a-b. Panel A: In response to the visual motion stimulus, the hearing aid user with excellent speech perception (1 dB SNR on the QuickSIN) shows activation restricted to occipital/cerebellar (visual) regions. Panel B: The hearing aid user with poor speech perception in noise (8 dB SNR on QuickSIN) shows activation in temporal (auditory) cortical regions, suggestive of cross-modal reorganization by vision. Adapted from Glick & Sharma (2017).39
Taken together, these individual case studies highlight how cross-modal reorganization may be one factor underlying the variability in patient performance outcomes after audiological intervention. Current research in our laboratory is examining these brain changes on larger groups of patients, before and after hearing aid use.
Discussion
With a better understanding of how the brain changes, secondary to hearing loss, we may be able to develop tools to detect early neural changes, before these changes can be seen on a conventional audiogram. Although our research findings are interesting and suggestive of the plasticity the brain is capable of in the presence of hearing loss, we hope to gain a better understanding of how hearing loss is linked to different aspects of neural function, such as cognition, speech perception, and depression.
Given the growing body of evidence linking hearing loss to cognitive decline, research in this area has the potential for an immense impact.59-61 This research may also help us predict which patients may perform well with hearing aids and which might do better with a cochlear implant—and, importantly, which patients need additional training or support. With future research, we may also be able to develop new and better aural rehabilitation and “brain-training” programs to maximize behavioral outcomes for patients with hearing loss.
Brain-based biomarkers may eventually help revolutionize how we treat and manage patients with hearing loss.
Acknowledgements
This research is supported by the National Institutes of Health.
Citation for this article: Sharma A, Glick H. Cortical neuroplasticity in hearing loss: Why it matters in clinical decision-making for children and adults. Hearing Review. 2018;25(7):20-24.
Correspondence can be addressed to Dr Sharma at: [email protected]
References
-
Kral A, Tillein J, Heid S, Hartmann R, Klinke R. Postnatal cortical development in congenital auditory deprivation. Cerebral Cortex. 2005;15(5):552-562.
-
Kral A, Sharma A. Developmental neuroplasticity after cochlear implantation. Trends Neurosci. 2012;35(2):111-122.
-
Ponton CW, Eggermont JJ. Of kittens and kids: Altered cortical maturation following profound deafness and cochlear implant use. Audiol Neurotol. 2001;6:363-380.
-
Sharma A, Dorman MF. Central auditory development in children with cochlear implants: Clinical implications. Advances Otorhinolaryngol. 2006;64:66-88.
-
Sharma A, Gilley PM, Dorman MF, Baldwin R. Deprivation-induced cortical reorganization in children with cochlear implants. Int J Audiol. 2007;46(9):494-499.
-
Kral A, Eggermont JJ. What’s to lose and what’s to learn: Development under auditory deprivation, cochlear implants and limits of cortical plasticity. Brain Research Rev. 2007;56(1):259-269.
-
Sharma A, Dorman MF, Spahr AJ. A sensitive period for the development of the central auditory system in children with cochlear implants: Implications for age of implantation. Ear Hear. 2002;23(6):532-539.
-
Sharma A, Spahr A, Dorman M, et al. Early cochlear implantation in children allows normal development of central auditory pathways. Annals in Otol Rhinol Laryngol. 2002;111(5):38-41.
-
Sharma A, Martin K, Roland P, et al. P1 latency as a biomarker for central auditory development in children with hearing impairment. J Am Acad Audiol. 2005;16:564-573.
-
Sharma A, Dorman MF, Kral A. The influence of a sensitive period on central auditory development in children with unilateral and bilateral cochlear implants. Hear Res. 2005;203(1-2):134-143.
-
Manrique M, Cervera-Paz FJ, Huarte A, Molina M. Advantages of cochlear implantation in prelingual deaf children before 2 years of age when compared with later implantation. Laryngoscope.2004;114(8):1462-1469.
-
Kirk KI, Ying E, Miyamoto RT, et al. Effects of age at implantation in young children. Ann Otol Rhinol Layrngol.2002;111[Suppl 5]:69-73.
-
Geers AE. Factors influencing spoken language outcomes in children following early cochlear implantation. Advances Otorhinolaryngol. 2006;64:50-65.
-
Niparko JK, Tobey EA, Thal DJ, et al. Spoken language development in children following cochlear implantation. J Am Med Assn. 2010;303(15):1498-1506.
-
Tajudeen BA, Waltzman SB, Jethanamest D, Svirsky MA. Speech perception in congenitally deaf children receiving cochlear implants in the first year of life. Otol Neurotol.2010;31(8):1254-1260.
-
Meinzen-Derr J, Wiley S, Grether S, Choo DI. Language performance in children with cochlear implants and additional disabilities. Laryngoscope.2010;120(2):405-413.
-
Birman CS, Elliott EJ, Gibson WPR. Pediatric cochlear implants: Additional disabilities prevalence, risk factors, and effect on language outcomes. Otol Neurotol. 2012;33(8):1347-1352.
-
Wiley S, Jahnke M, Meinzen-Derr J, Choo D. Perceived qualitative benefits of cochlear implants in children with multi-handicaps. Int J Ped Otorhinolaryngol. 2005;69(6):791-798.
-
Wiley S, Meinzen-Derr J, Grether S, Choo DI, Hughes ML. Longitudinal functional performance among children with cochlear implants and disabilities: A prospective study using the Pediatric Evaluation of Disability Inventory. Int J Ped Otorhinolaryngol.2012;76(5):693-697.
-
Berlin CI, Hood LJ, Morlet T, et al. Multi-site diagnosis and management of 260 patients with auditory neuropathy/dys-synchrony (auditory neuropathy spectrum disorder). Int J Audiol.2010;49(1):30-43.
-
Kirkim G, Serbetcioglu B, Erdag TK, Ceryan K. The frequency of auditory neuropathy detected by universal newborn hearing screening program. Int J Ped Otorhinolaryngol. 2008;72(10):1461-1469.
-
Talaat HS, Kabel AH, Samy H, Elbadry M. Prevalence of auditory neuropathy (AN) among infants and young children with severe to profound hearing loss. Int J Ped Otorhinolaryngol. 2009;73(7):937-939.
-
Vignesh SS, Jaya V, Muraleedharan A. Prevalence and audiological characteristics of auditory neuropathy spectrum disorder in pediatric population: A retrospective study. Indian J Otolaryngol Head Neck Surg. 2016;68(2):196-201.
-
Starr A, Picton TW, Sininger Y, Hood LJ, Berlin CI. Auditory neuropathy. Brain. 1996;119(3):741-753.
-
Deltenre P, Mansbach AL, Bozet C, et al. Auditory neuropathy with preserved cochlear microphonics and secondary loss of otoacoustic emissions. Audiology. 1999;38(4):187-195.
-
Rance G, Cone-Wesson B, Wunderlich, J, Dowell R. Speech perception and cortical event related potentials in children with auditory neuropathy. Ear Hear. 2002;23(3):239-253.
-
Corrales CE, Oghalai JS. Cochlear implant considerations in children with additional disabilities. Current Otorhinolaryngol Reports. 2013;1(2):61-68.
-
Fitzpatrick EM, Johnson E, Durieux-Smith A. Exploring factors that affect the age of cochlear implantation in children. Int J Ped Otorhinolaryngol.2011;75(9):1082-1087.
-
Kilbaugh TJ, Friess SH, Raghupathi R, Huh JW. Sedation and analgesia in children with developmental disabilities and neurologic disorders. Int J Ped. 2010;Article ID 189142.
-
Wang X, Xu Z, Miao C-H. Current clinical evidence on the effect of general anesthesia on neurodevelopment in children: An updated systematic review with meta-regression. PLoS One.2014;9(1):e85760.
-
Sharma A, Glick H, Campbell J, Biever A. Central auditory development in children with hearing impairment: Clinical relevance of the P1 CAEP biomarker in children with multiple disabilities. Hearing, Balance and Communication. 2013;11(3):110-120.
-
Campbell J, Cardon G, Sharma A. Clinical application of the P1 cortical auditory evoked potential biomarker in children with sensorineural hearing loss and auditory neuropathy spectrum disorder. Sem Hear. 2011;32(2):147-155.
-
Sharma A, Campbell J, Cardon G. Developmental and cross-modal plasticity in deafness: Evidence from the P1 and N1 event related potentials in cochlear implanted children. Int J Psychophysiol.2015;95(2):135-144.
-
Sharma A, Cardon G. Cortical development and neuroplasticity in auditory neuropathy spectrum disorder. Hear Res. 2015;330(B):221-232.
-
Sharma A, Cardon G, Henion K, Roland P. Cortical maturation and behavioral outcomes in children with auditory neuropathy spectrum disorder. Int J Audiol.2011;50(2):98-106.
-
Cardon G, Campbell J, Sharma A. Plasticity in the developing auditory cortex: Evidence from children with sensorineural hearing loss and auditory neuropathy spectrum disorder. J Am Acad Audiol. 2012;23(6):396-495.
-
Gilley PM, Sharma A, Dorman M, Finley CC, Arunachalam SP, Martin K. Minimization of cochlear implant stimulus artifact in cortical auditory evoked potentials. Clin Neurophysiol. 2006;117(8):1772-1782.
-
Lomber SG, Meredith MA, Kral A. Cross-modal plasticity in specific auditory cortices underlies visual compensations in the deaf. Nature Neurosci. 2010;13:1421-1427.
-
Glick H, Sharma A. Cross-modal plasticity in developmental and age-related hearing loss: Clinical implications. Hear Res. 2017;343:191-201.
-
Sharma A, Glick H. Cross-modal re-organization in clinical populations with hearing loss. Brain Sci.2016;6(1).
-
Campbell J, Sharma A. Visual cross-modal re-organization in children with cochlear implants. PLoS One. 2016;11(1):e0147793.
-
Campbell J, Sharma A. Cross-modal re-organization in adults with early stage hearing loss. PLoS One.2014;9(2):e90594.
-
Sharma A, Glick H, Campbell J, Torres J, Dorman M, Zeitler DM. Cortical plasticity and reorganization in pediatric single-sided deafness pre- and postcochlear implantation: A case study. Otol Neurotol. 2016;3(2)7:e26–e34.
-
Sandmann P, Dillier N, Eichele T, et al. Visual activation of auditory cortex reflects maladaptive plasticity in cochlear implant users. Brain. 2012;135(2):555-568.
-
Doucet ME, Bergeron F, Lassonde M, Ferron P, Lepore F. Cross-modal reorganization and speech perception in cochlear implant users. Brain. 2006;129(12):3376-3383.
-
Stropahl M, Plotz K, Schönfeld R, et al. Cross-modal reorganization in cochlear implant users: Auditory cortex contributes to visual face processing. Neuroimage. 2015;121:159-170.
-
Strelnikov K, Marx M, Lagleyre S, Fraysse B, Deguine O, Barone P. PET-imaging of brain plasticity after cochlear implantation. Hear Res.2015;322:180-187.
-
Strelnikov K, Rouger J, Demonet J-F, et al. Does brain activity at rest reflect adaptive strategies?? Evidence from speech processing after cochlear implantation. Cerebral Cortex. 2010:20(5):1217-1222.
-
Strelnikov K, Rouger J, Demonet J-F, et al. Visual activity predicts auditory recovery from deafness after adult cochlear implantation. Brain. 2013;136(12):3682–3695.
-
Kim M-B, Shim H-Y, Jin SH, et al. Cross-modal and intra-modal characteristics of visual function and speech perception performance in postlingually deafened, cochlear implant users. PLoS One. 2016;11(2):e0148466.
-
Heggdal POL, Brännström J, Aarstad HJ, Vassbotn FS, Specht K. Functional-structural reorganisation of the neuronal network for auditory perception in subjects with unilateral hearing loss: Review of neurimaging studies. Hear Res. 2016;332:73-79.
-
Kitterick PT, Lucas L, Smith SN. Improving health-related quality of life in single-sided deafness: A systematic review and meta-analysis. Audiol Neurotol. 2015;20[S1]:79-86.
-
Bess FH, Rothpletz A, Murphy JD. Children with unilateral sensorineural hearing loss. In: Newton V. Paediatric Audiological Medicine. 3rd ed. West Sussex, UK: Wiley;2009.
-
Van Zon A, Peters JPM, Stegeman I, Smit AL, Grolman W. Cochlear implantation for patients with single-sided deafness or asymmetrical hearing loss: A systematic review of the evidence. Otol Neurotol. 2015;36(2):209-219.
-
Campbell J, Sharma A. Compensatory changes in cortical resource allocation in adults with hearing loss. Frontiers Systems Neurosci. 2013;7(71):1-9.
-
Peelle JE, Troiani V, Grossman M, Wingfield A. Hearing loss in older adults affects neural systems supporting speech comprehension. J Neurosci.2011;31(35):12638-12643.
-
Wingfield A, Peelle JE. The effects of hearing loss on neural processing and plasticity. Frontiers Systems Neurosci.2015;9(35):
-
Schorr EA, Fox NA, van Wassenhove V, Knudson EI. Auditory-visual fusion in speech perception in children with cochlear implants. Proc Nat Acad Sci USA. 2005;102(51):18748-18750.
-
Lin FR, Ferrucci L, An Y, et al. Association of hearing impairment with brain volume changes in older adults. Neuroimage. 2014;90:84-92.
-
Lin FR, Ferrucci L, Metter EJ, An Y, Zonderman AB, Resnick SM. Hearing loss and cognition in the Baltimore Longitudinal Study of Aging. Neuropsychol. 2011;25(6):763-770.
-
Lin FR, Metter EJ, O’Brien RJ, Resnick SM, Zonderman AB, Ferrucci L. Hearing loss and incident dementia. Arch Neurol. 2011;68(2):214-220.
MORE ON “AUDIOLOGY & NEUROSCIENCE” from this special July 2018 edition of The Hearing Review:
Clinical Speech Audiometry in the Age of the AERP, by James Jerger, PhD.
Cortical Neuroplasticity in Hearing Loss: Why It Matters in Clinical Decision-Making for Children and Adults, by Anu Sharma, PhD, and Hannah Glick, AuD.
Effects of Amplification on Cortical Electrophysiological Function, by Sridhar Krishnamurti, PhD, and Larry Wise, AuD.
Cochlear implants: Considerations Regarding the Relationship between Cognitive Load Management and Outcome, by Edward Overstreet, PhD, and Michel Hoen, PhD.
Dementia Screening: A Role for Audiologists, by Douglas L. Beck, AuD, Barbara R. Weinstein, PhD, and Michael Harvey, PhD, ABPP.